Romancing the stone: DMSE researchers crack magnetic garnet mystery
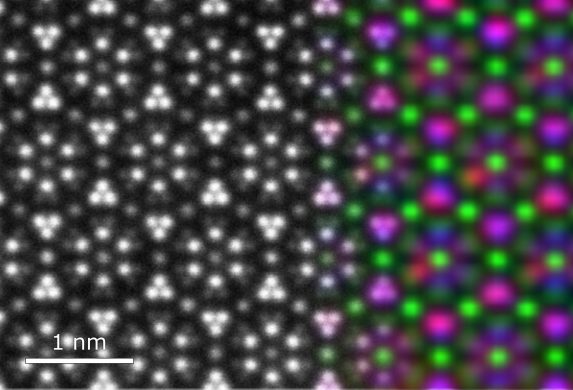
Scientists love a good mystery—it keeps them querying, testing, changing variables, and trying again. Sometimes a mystery lingers for decades, outlasting technological limitations—and setting the stage for a scientific breakthrough.
Such was the challenge Allison Kaczmarek and her colleagues faced four years ago. A graduate student in MIT’s Department of Materials Science and Engineering (DMSE), Kaczmarek set out to uncover why the magnetic properties of garnet crystal thin films were so peculiar.
Artificial garnets, synthesized in labs by scientists, are similar to the gemstone but are made with iron instead of silicon, making them magnetic. Typically, these crystals are isotropic, meaning their magnetic behavior doesn’t vary much no matter which way they’re positioned. But when the same magnetic garnets were grown as thin films for a now obsolete technology called bubble memory, their magnetic properties varied dramatically in different directions. This variation in thin films presented a compelling puzzle for researchers to solve.
Thermodynamics pioneer Herbert Callen attempted to unravel the phenomenon, known as growth-induced anisotropy, in 1971. He theorized that atoms of elements added during the growth process arranged themselves in a certain way, prompting the unusual properties. But at the time, the technology needed to observe what was happening at the atomic level didn’t yet exist, so Callen’s theory remained just that.
“Researchers didn’t really have a way to explain it other than this unproven theory,” Kaczmarek said. “So for a long time, they were just like, ‘Yeah, it must be that the atoms are ordering.’”
Until now. After more than 50 years, Kaczmarek and her advisors, Professors Geoffrey Beach and Caroline Ross, have confirmed Callen’s theory, in a recent Nature Communications paper. Using advanced imaging and analysis techniques, the researchers showed that in artificial garnet films made from rare-earth elements europium and thulium, the europium and thulium atoms arranged themselves in patterns that cause directionally dependent magnetism.
The findings suggest that by controlling the arrangement of atoms in these materials, researchers can fine-tune their properties, which could lead to the development of magnetic devices such as superfast memory technologies. These advancements could impact fields such as data storage, advanced electronics, and medical imaging.
Other authors of the paper include DMSE alum Ethan Rosenberg; graduate students Yixuan Song, Kevin Ye, and Gavin Winter; research specialist Aubrey Penn; and Associate Professor Rafael Gómez-Bombarelli.
The making of a mystery
The quest to explain the unusual magnetic properties of garnet thin films began with Rosenberg, who started growing these films before finishing his PhD in 2021, when Kaczmarek was a first-year student. Though he couldn’t fully explore them, his efforts set the stage for future discoveries.
“He had this crazy idea, ‘Oh, maybe we should try to look directly at ordering in these materials,” Kaczmarek said. “Now I’m in my fourth year and it’s just wrapping up. So it’s been a long journey.”
Rosenberg, and then Kaczmarek, started by revisiting research from the 1970s, when materials researchers made films of artificial garnet crystals for bubble memory, a data storage technology.
Thin films are layers of material deposited onto a substrate, usually a flat surface made of glass or another material. Researchers make them to study and optimize their properties, enabling advancements in various technologies. Bubble memory relied on thin films of magnetic material to hold “bubbles,” which stored information.
“They’d have these chips with the garnet material inside, and they’d have ways of creating these bubbles, which are magnetic domains, and moving them around—that’s how they stored data,” explained Ross, the Ford Professor of Engineering in DMSE. Since the bubbles needed to be stable as they were moved around to read, write, or store data, their magnetization had to point along particular directions, making lab-grown garnet films ideal for the task.
The thin-film production process is key to the material’s unusual properties. Researchers have been making magnetic garnets since the mid-20th century for microwave technology, used in radar systems and communication devices, and for magneto-optical devices in sensors and laser technology. But unlike their thin-film counterparts, the magnetism in three-dimensional garnet crystals does not have a preferred direction.
At the time, the methods for growing thin films differed from today’s sophisticated deposition processes, which involve applying nanometer-thin layers of material to a surface in a vacuum chamber. To make garnet thin films, researchers used to heat raw materials like rare-earth and iron oxides until they melted and then let them grow on a garnet substrate.
Two researchers making garnet thin films, Allan Rosencwaig and W. J. Tabor of Bell Laboratories, noticed that atoms of various elements they used had integrated into the material’s crystal structure—the repeating arrangement of atoms in gemstones, metals, and ceramics. So they consulted with Callen, to understand the phenomenon better. Callen was renowned for his expertise in statistical mechanics and thermodynamics, key fields for understanding complex atomic-level phenomena.
Callen proposed that the specific conditions during the thin-film growth process—temperature, composition, and the surface they were grown on—led to the atoms arranging themselves in specific patterns.
He pointed out that as the atoms joined the garnet material, the “sites,” or locations where they attach, have different shapes. For example, some sites might be triangular while others look square. Callen theorized that atoms of different elements prefer specific sites in the crystal structure, and this selective arrangement is what makes the material’s magnetism directionally dependent.
This atomic ordering was less pronounced in three-dimensional garnet crystals because the atoms attached to various sites in the crystal structure at once, so they didn’t arrange themselves in the same distinct patterns.
“That’s how this theory that there’s ordering started, but there hasn’t been real observation of the order until now,” Kaczmarek said.
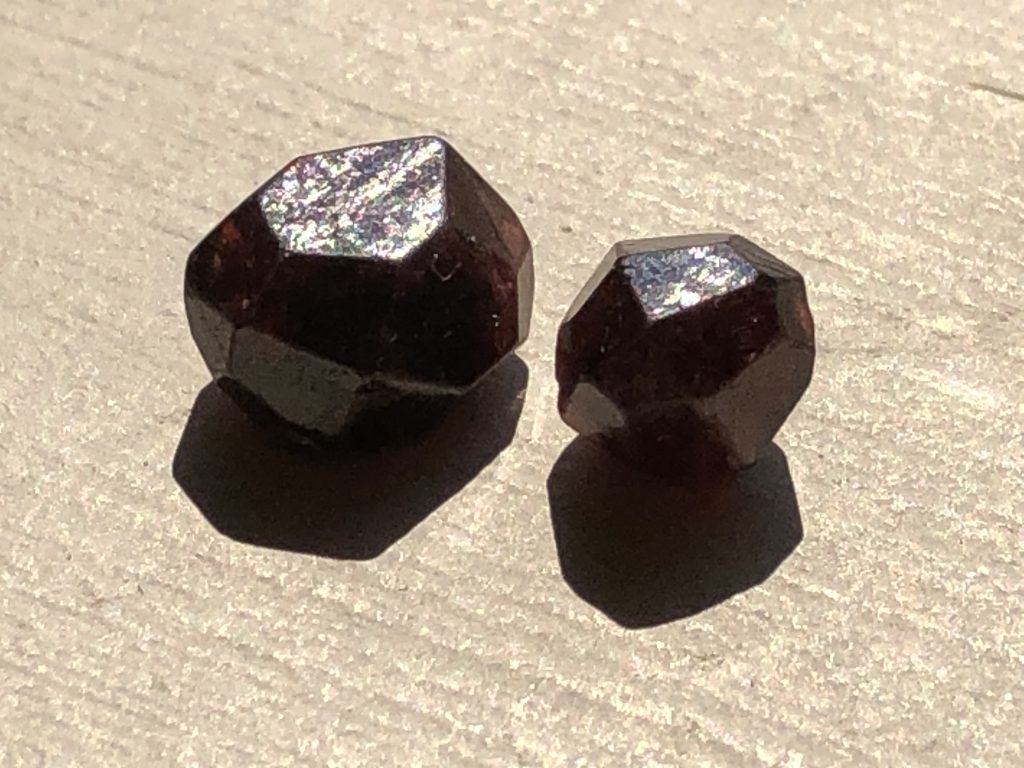
Piecing it together
That’s partly because researchers spent less time growing and thinking about garnet thin films. Bubble memory fell out of favor in the late ’70s, as hard disk and solid-state drives got faster and their storage capacity increased.
Despite the waning interest in studying the material’s properties for bubble memory, Kaczmarek saw an untapped opportunity to revisit and solve the longstanding puzzle. Without a deeper understanding of the properties, researchers could miss out on discovering advanced magnetic materials for countless new applications.
She sought to validate Callen’s theory, re-creating the old experiments with the aid of modern technology. She began by preparing garnet samples using an advanced thin-film technique called pulsed laser deposition, “a very fun way to make a material,” Kaczmarek said. It works by firing a laser at a target material in a vacuum chamber, vaporizing it. The material is then collected onto a substrate.
The films Kaczmarek made were ultrathin, just a few nanometers thick, allowing her to easily “tune” the composition of the material and study the atomic arrangement in the crystals. She chose europium and thulium for the different sizes of their atoms, ensuring that they would separate into different sites in the crystal structure.
Rosenberg’s initial work in growing these films laid a crucial foundation. Kaczmarek now had the tools and the setup to further investigate and solve the puzzle.
“We grew the films, and we were immediately getting the same results as people 50 years ago”—the material had the same unusual magnetic properties, Kaczmarek said. “But we still didn’t have the visuals, the images of these atoms.”
Unlike Callen in the early ’70s, Kaczmarek could visualize atoms doing what researchers long thought they were doing, with scanning transmission electron microscopy (STEM). This powerful technique focuses a beam of electrons through a material sample, collecting various signals to form an image.
Using STEM in conjunction with atomic resolution spectroscopy, which can single out individual atoms, Kaczmarek could determine which elements were present in the garnet films she made and at what proportions.
But it was no mean feat—since the theory she was trying to confirm predated the tools she was using, there were no protocols for indexing and interpreting data.
“None of the characterization routes had been developed,” Kaczmarek said. “So I really had to inspect the crystal structure carefully and see where can I actually find information that’s useful to me, and where do I have to look somewhere else, or how do I know that what I’m looking at is showing me this or not?”
After identifying specific patterns of atoms indicated in Callen’s theory, Kaczmarek quickly saw evidence that confirmed it. She described her joy when she first saw the rare-earth atoms occupying the sites Callan predicted, which was right around Thanksgiving in 2023.
“We had just seen this image that clearly showed the europium are here, and the thulium are here. And of course, I immediately sent it to Geoff and Caroline, and I can’t forget, Geoff’s response was, ‘Happy Thanksgiving—I’m really gobbling this up!’”
In the paper, Kaczmarek renames the automatic ordering of the elements that form the garnet crystals “magnetotaxial anisotropy”—combining the familiar term for magnetic materials with the Greek “taxis,” meaning arrangement or order. She found the old term, “growth-induced anisotropy,” strongly associated with bubble-memory garnets, outdated and limiting.
“This is an important phenomenon, and I don’t think that it’s only a garnet thing. I think that it must exist elsewhere as well. It must exist in other materials,” Kaczmarek said.
Bethanie Stadler, a professor of electrical and computer engineering at the University of Minnesota who was not involved in the study, underscored the significance of focusing on complex oxides, a broad category that includes perovskites, popular in materials research because of their applications in solar cells, lasers, and more. Garnets, complex oxides with a far more intricate crystal structure, offer a richer potential for discovery.
“You could put half the periodic table into the garnet structure if you get the cation ratios right,” said Stadler, a DMSE alum, referring to the positively charged ions that occupy sites in the crystal. “If you can solve the mystery for garnets, you can apply these insights to other complex oxide structures.”
Stadler also noted that the study’s findings on atomic ordering and anisotropy might lead to new ways to control how ions are arranged in crystals, which could affect their properties.
“I find this study interesting because it is likely to get readers thinking of new ways to control anisotropy. For garnets, ‘growth-induced’ anisotropy is a term that has been used like a generic diagnosis, so clarifying its origins is important for engineering new technologically important properties.”
Crystallizing possibilities
Now that the theory that growing artificial garnets causes directionally dependent magnetism is confirmed, researchers have a new “knob” to fine-tune the material’s magnetic properties, Kaczmarek said. By adjusting growth conditions—such as deposition speed, temperature, and pressure—they can control the degree of anisotropy, tailoring materials for specific applications.
“The processing will inherently change the end properties of our material,” Kaczmarek said.
Such precision opens the door to advanced magnetic devices and technologies that could
transform data storage, computing, and medical imaging. One key focus is spintronics, a new technology that leverages the “spin,” or momentum, of electrons for ultrafast data processing, lower power consumption, and greater data storage capacity.
To support these applications, Ross is working on favoring out-of-plane magnetization in garnets and related complex oxide materials. This property, in which a material’s magnetism is aligned perpendicular to its surface, is crucial for spintronic and magneto-optical devices, which can be used for data storage, modulators, or sensors.
“We’re hoping that by taking advantage of this phenomenon, this magnetotaxial anisotropy, we can make materials which will be ideal for high-speed magnetic memory and magneto-optical and photonic devices.”
Ross wants to push the concept further still, creating garnet films with the desired properties, and transferring them to silicon substrates.
“Everyone loves silicon. You can build your whole circuit on silicon, but you can’t grow garnet on silicon, at least not easily,” Ross said. “An argument is to grow the garnet separately, on a garnet substrate, and then peel it off and transfer it.” Affixing it to silicon, the foundation of most electronic devices, would incorporate the garnet film’s unique properties into mainstream electronics.
Ross’s team is working with Professor Jeehwan Kim of MIT’s Department of Mechanical Engineering and DMSE on this thin-film transfer technology, “a hot topic right now,” she says.
Stadler highlighted Kaczmarek’s rebranding of growth-induced anisotropy with the term “magnetotaxial,” suggesting it could inspire researchers to explore other ways of inducing this property.
“I think the new term does start people thinking, ‘What, besides the growth surface, could introduce an anisotropy like that?’” Stadler said. “It’s going to be really fun to see what comes next for complex oxide anisotropy.”
Beyond the applications, the “coolest thing” about the study is that it solved a 50-year-old mystery, said Ross. Her students dusted off an unconfirmed theory, applied modern experimental techniques, and closed this once-cold case.
“You think of stuff that happened before your students were born and they’ve proven it,” Ross said. “That’s very cool.”
This research was supported by the National Science Foundation’s Graduate Research Fellowship Program and the National Science Foundation Division of Materials Research.